CRISPR and the future of gene editing
BIOTECHNOLOGY
An insight into this powerful gene editing technology and what it could mean
for science and society.
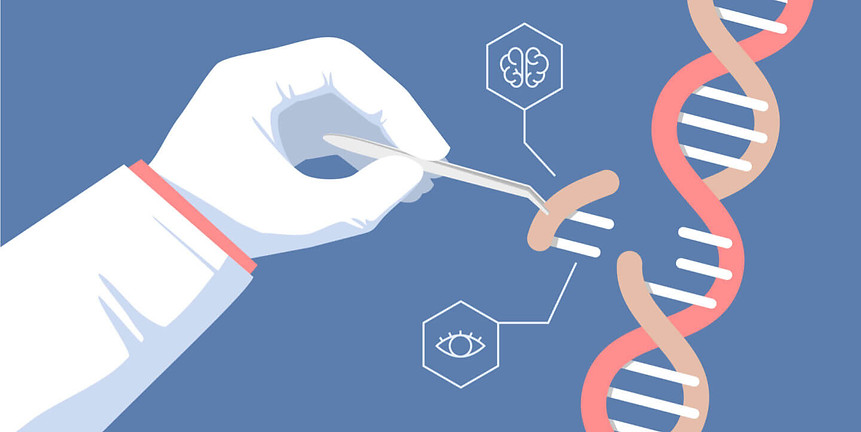
By Matt Warren
13th February 2018


A powerful DNA editing technology is poised to revolutionise molecular biology and our relationship with our genes. From curing genetic diseases to creating designer babies, this technology holds great promise, but is not without dangers. Here’s what you need to know about CRISPR and its applications to gene editing.
​​
The story begins in 1987, when researchers first reported the discovery of strange patterns in the genomes of E. coli bacteria. They observed multiple copies of a short repeating DNA sequence, each 30 base pairs long and separated by seemingly random sequences called spacers (see diagram below). First characterised by Francisco Mojica, these configurations were dubbed “clustered regularly interspaced short palindromic repeats” – CRISPR (pronounced “crisper”) for short.
​
​
​
​​
​​
​
It was later discovered that the spacer sequences matched snippets from the genomes of bacteriophages – viruses that infect bacteria. Researchers also noticed that whenever these CRISPR sequences appeared in the genome, a group of genes – later named Cas genes for CRISPR-associated proteins – always tagged along nearby. In response to these findings Mojica correctly hypothesized that this CRISPR-Cas structure forms part of the bacterial adaptive immune system, defending these microbes against viruses by storing some of their viral DNA. Carl Zimmer explains just how this works in Quanta:
​
​​
"As the CRISPR region fills with virus DNA, it becomes a molecular most-wanted gallery, representing the enemies the microbe has encountered. The microbe can then use this viral DNA to turn Cas enzymes into precision-guided weapons. The microbe copies the genetic material in each spacer into an RNA molecule. Cas enzymes then take up one of the RNA molecules and cradle it. Together, the viral RNA and the Cas enzymes drift through the cell. If they encounter genetic material from a virus that matches the CRISPR RNA, the RNA latches on tightly. The Cas enzymes then chop the DNA in two, preventing the virus from replicating."
​
​​
As the underlying mechanics of the CRISPR-Cas system emerged, the potential applications became apparent to scientists around the world. Their train of thought would have run something like this: Cas enzymes are nucleases, that is they cut DNA, and the RNA tells Casprecisely where to cut; by tailoring the RNA, a specific DNA sequence within any genome can, in theory, be targeted and cut with exclusivity. A dream come true for molecular biologists.
​
In the years that followed, further investigations from independent academic groups lead to the publishing of two pivotal research papers in the journals Science and PNAS. Using CRISPR-Cas9, a variation adapted from Streptococcus pyogenes bacteria, the remaining gaps in our understanding of the mechanism were filled, illuminating the path to using the CRISPR-Cas system as a genome editing tool.
​
Notably, these studies demonstrated that Cas9 introduces double-stranded breaks in target DNA at a precise position in the nucleotide sequence, and revealed that two small RNA molecules are involved: CRISPR RNA (crRNA) copied from the spacer and an additional, trans-activating CRISPR RNA (tracrRNA). These would later be fused into a single, synthetic RNA guide, simplifying the system into just two components.
​
It wasn’t long before researchers had tweaked this system for genome editing in eukaryotic cells, targeting animal and plant genomes. In 2013, Feng Zhang and his team at the Broad Institute of MIT and Harvard reported they had successfully used of CRISPR-Cas9 to cleave target DNA in human and mouse cells. This landmark study would feature the first use of CRISPR to edit human cells, but certainly not the last.​
​​
​
But hasn’t genome editing has been going on for decades? Why is CRISPR suddenly such a big deal?
​
It’s true – gene editing is nothing new. In fact, molecular biologists have been tinkering with our genomes since discovering how to use restriction enzymes to cut DNA in 1970. Unlike Cas9, however, these enzymes had evolved to function as DNA shredders rather than precision tools. Where restriction enzymes recognize a short sequence of bases in DNA, typically between four and eight bases, Cas9 and its cognate guide RNA have a recognition site around 20 bases long. With approximately three billion base pairs in the human genome, any sequence of four bases should theoretically appear over ten million times, whereas a specific 20 base sequence is unlikely to appear at all, that is, unless you know it’s there in the first place. This ensures that Cas9 only cuts where directed, and the DNA isn’t chopped to bits.
​
CRISPR is also more efficient than other tools in the genetic editing toolkit, namely transcription activator-like effector nucleases (TALENs) and zinc finger nuclease (ZFN). These technologies recognise longer stretches of DNA, offering even greater specificity over CRISPR, and a lower chance of off-target effects. There is, however, one major downside: each time a new DNA target sequence is used, a TALEN or ZFN protein must be custom-designed and built, often requiring multiple iterations before arriving at a variation that works. Cas9, by comparison, is readily available and the guide RNA can simply be designed and ordered online, all at relatively low cost.
​
Another reason for the excitement over CRISPR-Cas9 is its potential beyond just cutting DNA. When double strand breaks are introduced into DNA, natural repair mechanisms kick in, attempting to fix the break and restore the genome. This is usually achieved by non-homologous end joining (NHEJ), where the broken ends are simply resected, or by another mechanism called homology directed recombination (HDR). In the case of HDR, a DNA template is used to reconnect the broken strands and precisely repair the damage, copying from the template.
​
By providing a customised repair template while simultaneously cutting DNA using CRISPR, the natural repair pathways of the cell can be harnessed to insert a desired sequence of DNA, generating a precise change in the genome. Functioning like a molecular cut-and-paste, this system can be used to insert novel genes, repair defective ones, or knock-out a gene altogether. Editing the genome in this way can be used to bring about a variety of effects, with huge applications.
​​​
​
So what can CRISPR actually be used for, and is there cause for concern?
​
Among the biggest claims of CRISPR is its potential to eradicate genetic diseases. These are conditions resulting from mutated and dysfunctional copies of a gene, which can be inherited and passed down through subsequent generations. CRISPR could be used to remove the faulty genes, replacing them with healthy versions and effectively curing the disease. In 2016, an advisory panel at the National Institutes of Health (NIH) approved a proposal to use CRISPR on cells in a person, the first trial involving adult humans to be approved in the U.S.
​
The proposal outlines a plan to remove immune (T) cells from adults with melanoma, sarcoma or myeloma, edit them using CRISPR-Cas9 and then reinsert them, observing how humans respond to the therapy. However, the trial still hinges on additional approval from the medical organisations that will be conducting it, and the technology a long way from being used in a wider clinical population, with early evidence hinting that the process may not very efficient in adult human cells after all.
​
More recently, studies in U.S and China using CRISPR to edit genes in human embryos have shown greater promise. At Oregon Health & Science University, Shoukhrat Mitalipov and his team engineered embryos containing a specific defect known to cause a heart condition. He then used CRISPR to splice out the mutated gene when the embryos consisted of just one cell. When tested several days later, over 70% of the embryos showed no sign of the defective gene; the gene had been corrected in all of these cells.
​
Despite these successes, the process is fraught with ethical potholes and safety concerns. While curing a life-threatening inherited disease isn’t so controversial, using CRISPR to remove undesirable traits or enhance, say, ‘intelligence’ or athleticism, creating so called “super-babies”, becomes far more problematic. It is these ethical dilemmas that have led many leading biologists and bioethicists to call for an international moratorium on germline gene editing until all the issues surrounding CRISPR are better understood.
​
​
​
​
​
​
​
​
​
​
​
​​
Aside from ethical concerns, there are many technical obstacles between current research and a generation of 10-feet-tall Einsteins. Crucially, many human traits such as intelligence or height are polygenic, with tens, hundreds or even thousands of genes contributing to the phenotype, as well as myriad environmental factors. So, while CRISPR can almost certainly be used to edit one or a few disease-causing mutant genes, editing many genes in a single organism may well be beyond the practical capabilities of the technique, at least in its current technological state. Indeed, it may be many years before CRIPSR is used in clinics at all.
​
Meanwhile, CRISPR offers great promise among agricultural industries, and it is these sectors that Doudna suggests we should look for more immediate benefits of the technology. The efficiency and precision of CRISPR enables plant scientists to decipher the identity of genes and edit phenotypic characteristics far quicker than conventional breeding techniques, reducing the time to bring edited crops to market. These characteristics range from drought resistance to fruit flavour, and have already proved amenable to gene editing.
​
Moreover, as gene-edited plants do not contain foreign DNA added from other species, they do not qualify as genetically modified organisms (GMOs). Thus, the regulatory frameworks governing the use of these crops are less stringent and somewhat unclear, particularly in the U.S. In fact, the first genome-edited crop, a herbicide-resistant oilseed rape, has been planted in fields across America since 2015. Although this crop was not produced using CRISPR, it has set precedence for gene-editing in commercial agriculture, and CRISPR-engineered crops are tipped for release to U.S growers as early as 2021.
​
The applications of this technology are clearly profound, but, at this stage, shrouded in uncertainty. This technology could help rid humanity of genetic disease and food crises, but it is no biological panacea; its misuse could easily cause more problems than it might solve. “CRISPR is not a light on the nation, it’s a mirror,” said CrisprCon keynote speaker Greg Simon; in other words, it is a technology only as good as the people using it.

Among those concerned about CRISPR-Cas could be used is Jennifer Doudna, one
of the pioneers of the technology. Video: Ted.com